Micromechanics of Granular Materials
We are studying the micromechanics of granular materials – forces, fracture mechanics, rearrangements – using novel small-scale experimentation, theory, and modeling. Measurements are made using in-situ X-ray computed tomography (XRCT), 3D X-ray diffraction (3DXRD), and acoustic emissions (AE). This research will transform our basic understanding of, and ability to model, frictional 3D granular materials and similar amorphous systems such as colloids, gels, and emulsions in a variety of environments.
Students: Dr. Chongpu Zhai (Previous JHU Postdoc), Dr. Ghassan Shahin (Previous JHU Postdoc), Kwangmin Lee (Current JHU Ph.D. student), Nahuel Albayrak (AEOP REAP Summer Intern)
Current sponsor: U.S. National Science Foundation, CAREER Award (2020 – 2025).
Previous sponsor: Lawrence Livermore National Laboratory (LDRD), (2016 – 2018).
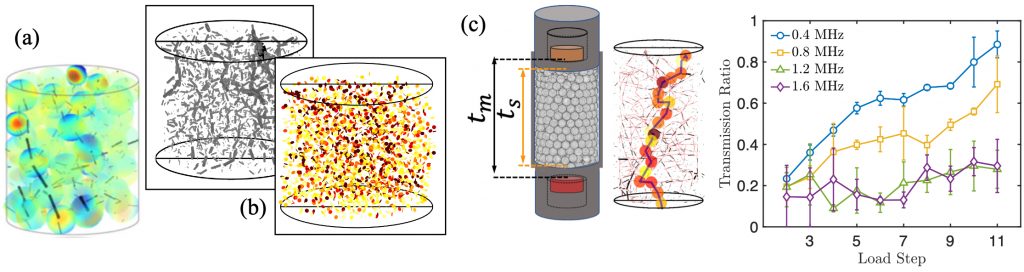
(a) Inter-particle forces in 3D from in-situ XRCT and 3DXRD measurements (see Hurley, et al. 2016 in Phys. Rev. Lett.) (b) Inter-particle forces and slip distances between individual particles (see Zhai, et al. 2019 in JMPS). (c) Ultrasound wave transmission, schematic, wave path, and wave attenuation. (Zhai, et al. 2020 in PNAS).
Dynamic Experiments and Constitutive Models for Dynamic Penetration of Geomaterials
As part of the Materials Science in Extreme Environments (MSEE) University Research Alliance, our group is leading a research Focus Area on Geomaterial Constitutive Models, which aims to bring advanced experiments together with mechanism-based models to predict the response of geomaterials to rapid impact. The advanced experiments we will be performing include two-stage gas gun impact experiments into rocks and soils with in-situ X-ray and other diagnostics in HEMI’s HyFIRE facility, drop tower impact experiments in our laboratory, and dynamic compaction experiments using various drivers and in-situ X-ray diagnostics at synchrotron facilities. Read more about the MSEE URA here.
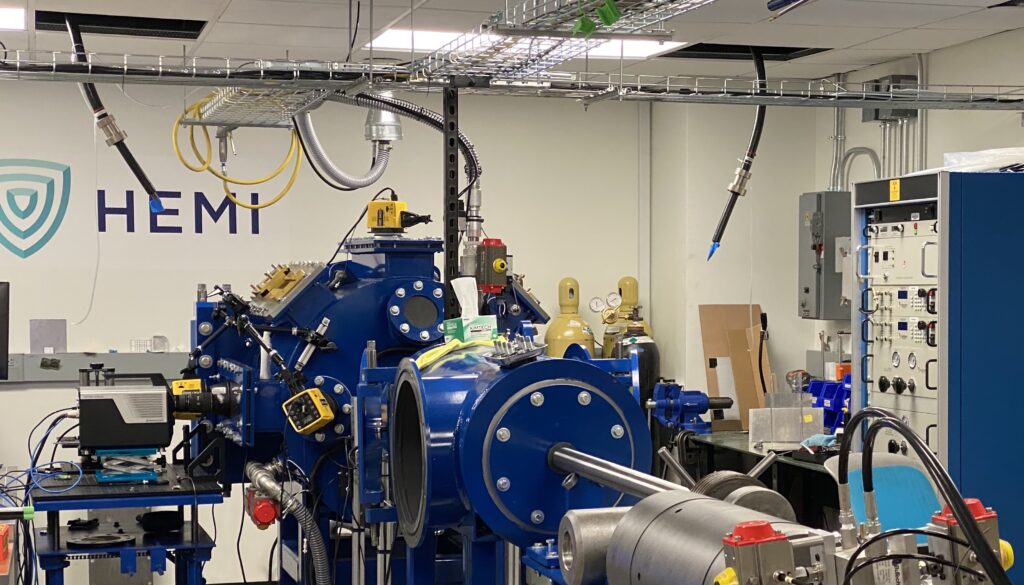
HyFIRE two-stage gas gun chamber, where many experiments supporting this research are performed.
Students: Brett Kuwik (Current JHU Ph.D. student), Ghassan Shahin (Previous JHU Postdoc)
Current sponsor: Materials Science in Extreme Environments (MSEE) University Research Alliance, Defense Threat Reduction Agency (DTRA) (2020 – 2025).
Breakage Mechanics and Granular Flow Modeling for Advanced Ceramics
We are developing constitutive models for granular flows that account for particle breakage and other micromechanical processes. These models are important for describing the zones of granulated ceramics that are generated during dynamic impact and fragmentation in body and vehicle armor applications.
Students: Adyota Gupta (Current JHU Ph.D. student, co-advised with KT Ramesh), Amartya Bhattacharjee (Current JHU Ph.D. student advised by Prof. Lori Graham-Brady), Dr. Mehmet Cil (Previous JHU Postdoc)
Previous sponsor: Army Research Laboratory, Materials in Extreme Dynamic Environments (MEDE) Collaborative Research Alliance.
3D Measurements and Models of Particle Kinematics, Structural Changes, and Stresses During Shock Compression of Granular Materials
We are developing measurements and theories to quantitatively describe the 3D particle kinematics, deformation, and porosity evolution during shock compression of granular materials. Experimentally, we are developing optimization algorithms that combine X-ray computed tomography (XRCT) with in-situ dynamic X-ray phase contrast imaging (XPCI) to infer 3D particle and pore kinematics. We are also developing a theoretical micromechanical model that relates the instantaneous stiffness of a granular packing to its microstructure.
Students: Sohanjit Ghosh (Current JHU Ph.D. student)
Current sponsor: Air Force Office of Scientific Research (AFOSR) Young Investigator Program (YIP) (2022 – 2025).
Previous sponsor: Partial Support From Army Research Laboratory, Materials in Extreme Dynamic Environments (MEDE) Collaborative Research Alliance (2019 – 2021).
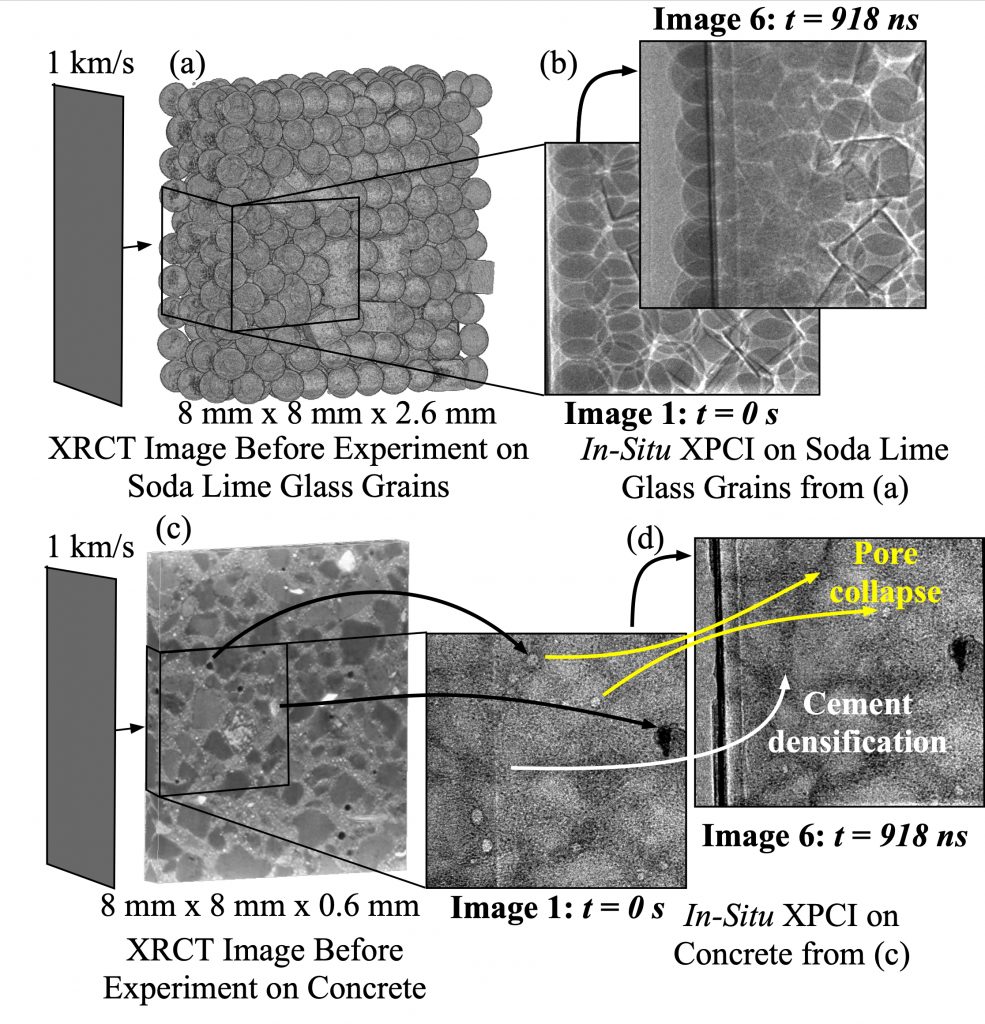
Examples of (a) XRCT image of a sample of soda lime glass spheres and cubes prior to (b) dynamic impact at 1 km/s; (c) XRCT image of a thin piece of cement prior to (d) dynamic impact at 1 km/s.
Cement Paste Micromechanics and Aggregate-Paste Interactions in Concrete
The goal of this project is to develop a quantitative understanding of whether and under what circumstances concrete mechanics can be predicted using continuum micromechanics theories. We are using in-situ X-ray measurements, digital volume correlation, modeling, and theory to understand inclusion interactions, fracture nucleation, and interfacial transition zone (ITZ) properties. The outcomes of this work will aid in the development and validation of mesoscale models for the prediction of material behavior in a variety of quasi-static and extreme loading conditions.
Students: Dr. M. Mohsin Thakur (Current JHU Postdoc), Dr. Ghassan Shahin (Current JHU Postdoc), Ziheng Wang (Former JHU M.S. Student)
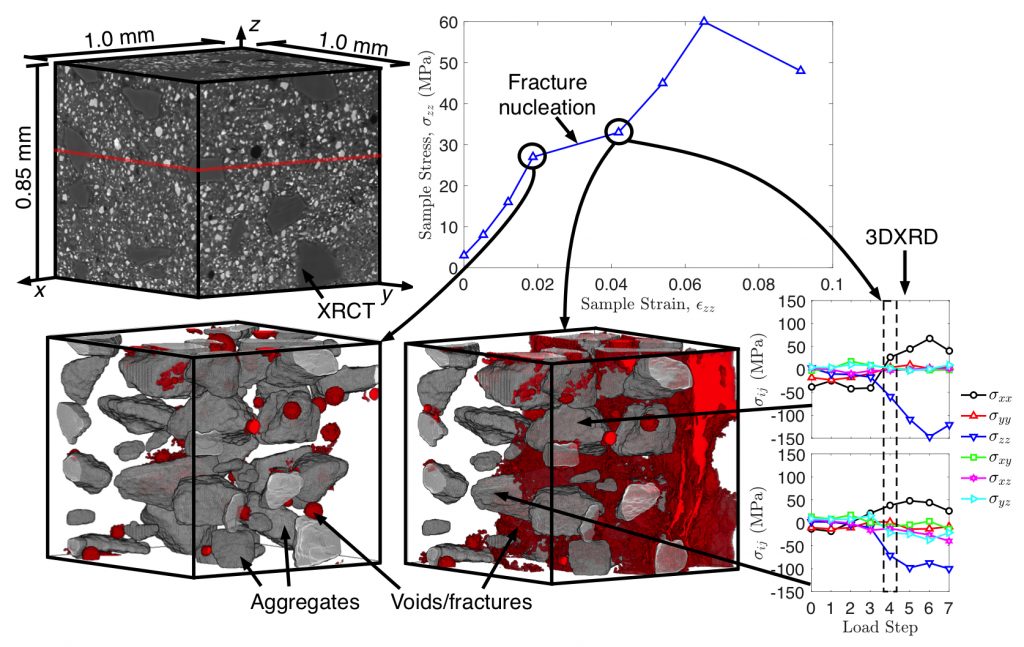
Fig. 1 – Results from an experiment performed at the Cornell High Energy Synchrotron Source C(HESS) employing XRCT to image microstructure and 3DXRD to measure stress tensors in each of 52 single-crystal quartz aggregate particles (i.e., sand grains) within a concrete specimen.
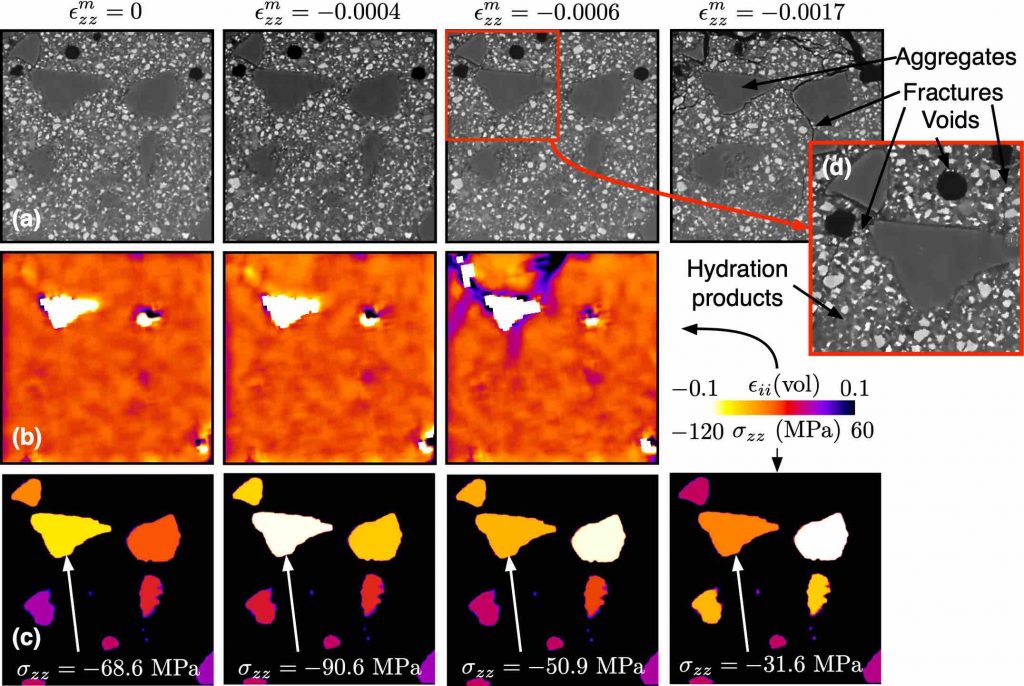
(a) Top row shows horizontal slices through the XRCT image shown in Fig. 1 at four macroscopic sample strain levels. (b) Middle row shows volumetric strain from DVC at three strain levels. Microcracks emerge at a vertical sample strain of -0.0006 (see inset in (d)) which are clearly detected with DVC. (c) Bottom row shows vertical stress tensor component (zz) of the aggregate whose ITZ is traversed by the microcrack at vertical sample strain of -0.0006. Ongoing work is devoted to making DVC measurements more quantitative by correcting time- and temperature-induced artifacts.
Current sponsor: U.S. National Science Foundation (2021 – 2024).